Cardiovascular stents, fluid flow and endothelial cell phenotype
Coronary heart disease is a major cause of death worldwide, and stenting has become one of the preferred therapies for treatment. In the USA alone about 650,000 stents are implanted yearly with 75% of these being drug eluting stents (DES). Unfortunately 1/3 of patients with bare metal stents (BMS) suffer from restenosis of the coronary artery, and about 1-2% of patients with DES suffer from in-stent thrombosis, leading to significant morbidity and mortality.
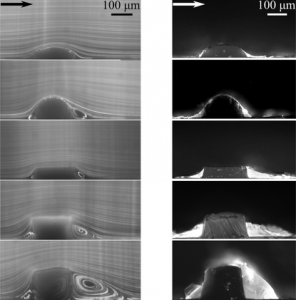
Left: Pathlines generated by 1 µm fluorescent particles in the vicinity of 5 different stent strut models under steady flow conditions demonstrate the formation of a range of recirculation zones by different geometries. Right: Representative images of proximal and distal fluorescent fibrin deposition near different stent strut models for pulsatile undisturbed flow inlet boundary condition.
Our research explores how blood flow perturbations caused by the stent design contribute to in-stent restenosis and thrombosis, studying the impact of the fluid forces on blood components and endothelial cells. We have introduced aerodynamic and fluid dynamic engineering principles into stent design, creating streamlined stent struts that differ from commercially available BMS and DES non-streamlined stent struts. Using stented coronary artery models exposed to coronary-like arterial fluid flows we examine: 1) the phenotype change of endothelial cells transcriptionally and/or translationally in the vicinity of nonstreamlined and streamlined stent struts, 2) endothelial cell migration (motility) under the influence of fluid flows created by the different stents struts, with implications to wound healing after coronary artery stenting, a marker of clinical success, 3) in the absence of endothelial cells, the role that stent geometries effect on coagulation and thrombus formation using freshly isolated blood.
Initial results have demonstrated that by introducing this novel engineering approach to stent design, the local blood flow field is changed yielding an anti-thrombotic endothelial cell phenotype and decreased thrombus formation, with implications for clinical success.
Future research planned includes determining the molecular basis for fluid flow-induced differences in endothelial cell migration, optimization of streamlined stent design, and pre-clinical studies of streamlined stents in coronary heart disease animal models.
Cerebral Hemodynamics
Five percent of adults are affected by brain aneurysms; one ruptures every 18 minutes. Forty percent of ruptures are fatal, while 2/3 of survivors suffer permanent neurologic injury. For this reason an understanding of their cause is imperative. Although there is a lot of research focuses on the prevention of rupture once an aneurysm has formed, there is little known about their initiation. We hypothesise that flow characteristics could have a significant impact in this initiation and we are therefore using computational fluid dynamics to simulate the fluid flow in patients with a unilateral aneurysm. By using medical images taken from such patients alongside digital tools, we are able to model the flow in the pre-aneurysm state and isolate flow properties which could be leading to their cause. Further to this computational work, we are experimentally testing these hypotheses using cutting edge 3D bioprinting and PIV techniques alongside our expertise in cell culturing. The project is therefore multidisciplinary, involving a range of experts from high performance computational simulation to molecular biology, in a collaborative effort involving researchers from all over the world.
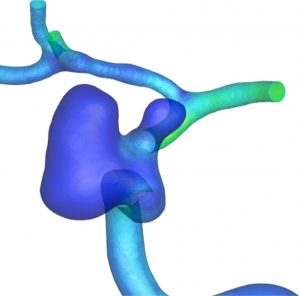
CFD simulations are undertaken on these patient-specific computational meshes in order to elucidate the potential flow-related causes of aneurysm, in this case specifically in the vasculature of the brain.
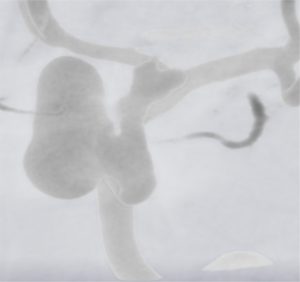
Typical raw data from an angiogram taken of a patient with a unilateral aneurysm. The blood vessels and aneurysm are more highly resolved than the surrounding tissue and bone in this type of imaging.
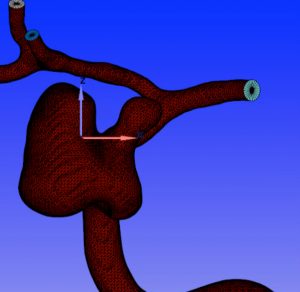
Raw outputs from medical imaging are processed into surface meshes through image segmentation. This removes all unwanted features and noise from the data and results in a clean geometry ready for simulation.
Lymphatic Fluid Flow and the Endothelium
In the lymphatic system, the role of fluid flow in development and disease is poorly understood. In collaboration with a team of cardiologists and vascular biologists, we have demonstrated that fluid flow serves as a physical stimulus inducing expression of genes required for lymphatic valve development.
Future research planned includes characterizing the interplay between lymphatic flow and cell transport in immunity, cancer metastasis, and lymphedema.